Breathing New Life into Treatment: The Revolutionary Promise of Inhaled Drug Delivery
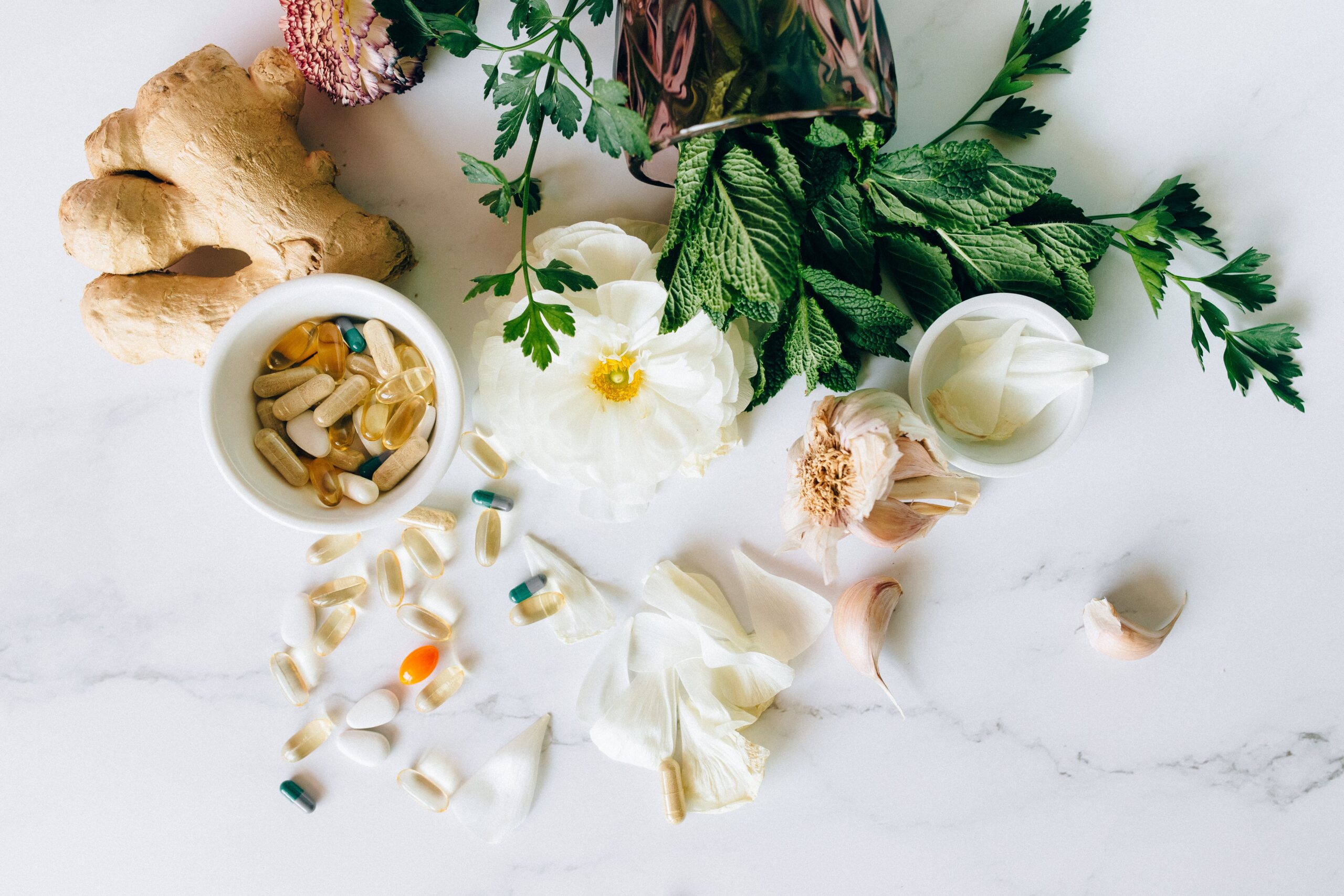
Inhaled drug delivery focuses on the direct delivery of therapeutic agents to the lungs via inhalation. This type of administration differs from traditional methods such as oral administration or administration by injection and takes advantage of the unique anatomy and physiology of the respiratory system to provide efficient and targeted treatment.
Its appeal lies in its non-invasive nature and potential for rapid onset of action, particularly in respiratory diseases. However, the use of inhaled drug delivery is not limited to treating lung-specific diseases. This is becoming increasingly important in systemic therapy, taking advantage of the large surface area and rich blood supply of the lungs to effectively absorb drugs into the bloodstream.
The technology behind inhaled drug delivery is complex, involving aerosol science, formulation chemistry, and lung biology. Factors such as particle size, formulation stability, and delivery efficiency must be carefully considered to ensure that therapeutic agents reach their intended site of action within the lung.
Inhaled drug delivery is therefore a promising but challenging milestone in modern medicine, offering potential benefits in terms of efficacy, safety, and patient compliance.
Inhalable RNA Therapeutics Delivery Methods
Inhalable RNA therapeutic delivery methods constitute an advanced paradigm in pharmaceutical biotechnology focused on the pulmonary delivery of ribonucleic acid (RNA) molecules for therapeutic purposes. This approach uses the respiratory system as the entry point for systemic drug delivery, specifically targeting the lung epithelium and alveolar macrophages, which are important sites of therapeutic intervention in respiratory diseases.
Aerosolization of RNA Molecules:
This involves the conversion of RNA therapeutics into aerosol form using nebulizers or metered-dose inhalers. The process must ensure the generation of aerosol droplets or particles of an optimal aerodynamic diameter, typically within the range of 1-5 micrometers, to facilitate deep lung deposition and avoid mucociliary clearance.
Bioavailability Enhancement:
Given the susceptibility of RNA molecules to enzymatic degradation in the respiratory tract, inhalable RNA therapeutics often incorporate molecular stabilization strategies. These might include the use of nucleotide modifications, such as 2′-O-methylation, to increase resistance against ribonucleases, thereby enhancing the stability and bioavailability of the RNA molecules post-inhalation.
Pulmonary Drug Delivery Systems:
These systems are designed to optimize the deposition of RNA therapeutics in the target regions of the lungs. Factors like particle size, density, shape, and hygroscopicity are meticulously engineered to ensure effective pulmonary distribution. The goal is to maximize alveolar deposition while minimizing oropharyngeal losses and exhalation of the therapeutic agent.
Cellular Uptake and Endosomal Escape:
Once deposited in the pulmonary tissue, RNA therapeutics must be efficiently taken up by target cells, typically through endocytosis. The design of RNA molecules or their carriers often includes mechanisms to facilitate endosomal escape, thereby ensuring the release of RNA into the cytoplasm where it can exert its therapeutic effect.
Immunogenicity Considerations:
Inhalable RNA therapeutics must be designed to minimize immune activation, particularly avoiding the stimulation of pattern recognition receptors like Toll-like receptors (TLRs), which can recognize RNA molecules as foreign and trigger inflammatory responses. This is critical to prevent adverse immunogenic effects and ensure patient safety.
By leveraging these advanced scientific principles, inhalable RNA therapeutics offer a novel, non-invasive route for the administration of RNA-based drugs, presenting significant potential in the treatment of a variety of diseases, particularly those affecting the respiratory system.
Inhaled RNA vaccine development
Inhaled RNA vaccine development represents a cutting-edge domain in molecular immunology and pharmaceutics, where the primary objective is to harness the potential of RNA molecules, particularly messenger RNA (mRNA), as immunogens. This modality of vaccine delivery leverages pulmonary administration to elicit robust immune responses against specific pathogens. Specific aspects involved in the development of inhaled RNA vaccines include:
mRNA Synthesis and Optimization:
The foundation of inhaled RNA vaccines is the synthetic mRNA, encoding the antigen of interest—often a viral protein. This mRNA is bioengineered using in vitro transcription (IVT) techniques. Modifications such as cap analog addition, incorporation of modified nucleotides like pseudouridine, and optimization of the 5’ and 3’ untranslated regions (UTRs) are crucial. These modifications enhance translational efficiency and stability while reducing innate immunogenicity.
Pulmonary Delivery Vectors:
Critical to the success of inhaled RNA vaccines is the development of suitable delivery vectors that can protect the mRNA from degradation in the respiratory tract. Lipid nanoparticles (LNPs) are predominantly used for this purpose. These LNPs encapsulate mRNA and facilitate its entry into the pulmonary epithelial cells via endocytosis. They are engineered for optimal size, charge, and lipid composition to ensure efficient pulmonary deposition and cellular uptake.
Mucosal Immune Activation:
The respiratory mucosa is rich in antigen-presenting cells (APCs) like dendritic cells and macrophages. Upon pulmonary administration, the mRNA within the vaccine is expressed in the epithelial cells and APCs, leading to the presentation of the encoded antigen on major histocompatibility complex (MHC) molecules. This antigen presentation is pivotal for activating both the mucosal and systemic immune responses, including the generation of antigen-specific T-cells and the production of neutralizing antibodies.
Immunologic Adjuvants:
In some formulations, adjuvants may be incorporated to further enhance the immunogenicity of the vaccine. These adjuvants can be designed to stimulate specific toll-like receptors (TLRs) or other pattern recognition receptors (PRRs) on immune cells, thereby potentiating the innate immune response and augmenting the adaptive immune response.
Aerosolization and Particle Engineering:
The process of aerosolizing the RNA vaccine for inhalation involves sophisticated technologies to create particles with optimal aerodynamic properties for deep lung deposition. Factors such as particle size, density, and hygroscopic behavior are finely tuned to ensure that the vaccine reaches the lower respiratory tract for effective immune stimulation.
Biosafety and Immunotolerance:
Ensuring the biosafety of inhaled RNA vaccines is paramount. This involves rigorous testing for potential off-target effects, innate immune activation, and the risk of insertional mutagenesis. Additionally, strategies to induce immunotolerance and prevent hypersensitivity reactions, especially in repeated dosing scenarios, are critical considerations in vaccine design.
The development of inhaled RNA vaccines is thus a highly interdisciplinary endeavor, requiring expertise in molecular biology, immunology, pharmaceutics, and aerosol science. This approach offers promising prospects, particularly in inducing robust local and systemic immunity against respiratory pathogens, and represents a significant advancement in the field of vaccinology.
Efficiency of RNA delivery via inhalation
The efficiency of RNA delivery via inhalation relies on the optimization of various parameters such as aerosol science, biophysics, and cellular biology to ensure the effective and targeted delivery of RNA molecules, particularly mRNA or siRNA, to the pulmonary system for therapeutic action. Critical scientific elements involved in enhancing the efficiency of RNA delivery via inhalation include:
Aerosol Dynamics and Deposition Mechanics:
The efficiency of RNA delivery through inhalation is largely contingent on the aerodynamic properties of the aerosolized particles. Parameters such as particle size, shape, density, and hygroscopicity are key determinants of the aerodynamic behavior and, consequently, the deposition pattern in the respiratory tract. Particles in the range of 1-5 micrometers in diameter are typically targeted to achieve deep lung deposition, bypassing the upper airway filtration mechanisms and facilitating alveolar delivery.
Pulmonary Barrier Penetration and Absorption:
The pulmonary epithelium, with its tight junctions and mucociliary clearance mechanism, presents a significant barrier to effective RNA delivery. Overcoming this involves strategies to enhance the penetration and absorption of RNA molecules across the epithelial barrier, such as the use of lipid nanoparticles (LNPs) or other carrier systems that can facilitate endocytosis and transcytosis of RNA into the target cells.
Stability and Bioavailability of RNA:
The susceptibility of RNA molecules to enzymatic degradation in the respiratory environment necessitates molecular stabilization strategies. Modifications such as 2′-O-methylation or incorporation of pseudouridine can increase the stability of RNA, thereby enhancing its bioavailability and half-life in the pulmonary system.
Cellular Uptake and Intracellular Trafficking:
Efficient cellular uptake and intracellular trafficking of RNA are paramount for successful therapeutic action. This involves the endocytosis of RNA-loaded nanoparticles by target cells and subsequent endosomal escape, ensuring the release of RNA into the cytoplasm where it can exert its intended function, be it protein synthesis (in the case of mRNA) or gene silencing (in the case of siRNA).
Immunogenicity and Tolerance:
The immune response to inhaled RNA therapies must be carefully managed. Minimizing the immunogenicity of RNA molecules and their carriers is essential to avoid unintended activation of the immune system, which could lead to inflammation or hypersensitivity reactions. At the same time, for RNA vaccines, controlled immune activation is desired to elicit a protective response.
Pharmacokinetic and Pharmacodynamic Profiling:
Understanding the pharmacokinetics (absorption, distribution, metabolism, and excretion) and pharmacodynamics (the biological effect and mechanism of action) of inhaled RNA is crucial. This involves the use of advanced imaging techniques and bioanalytical methods to track the distribution and persistence of RNA in the lungs and to correlate these with the therapeutic outcome.
Patient-Specific Factors:
Variability in lung physiology and pathology among patients can significantly impact the efficiency of RNA delivery via inhalation. Personalized approaches considering factors like lung capacity, disease state, and mucosal characteristics may be required to optimize delivery efficiency for individual patients.
Enhancing the efficiency of RNA delivery via inhalation requires a multidisciplinary approach, integrating advanced knowledge in aerosol technology, nanoparticle engineering, molecular biology, and pharmacology, aimed at overcoming the unique challenges posed by the pulmonary route of administration.
Get your weekly dose of industry news and announcements here, or head over to our Formulation Brand to catch up with the latest advances in manufacture and therapeutic delivery. If you’d like to learn more about our upcoming Formulation and Delivery US event, click here to download an agenda or register your interest.