Understanding the Capabilities of 3D Bioprinting for Cell and Gene Therapy
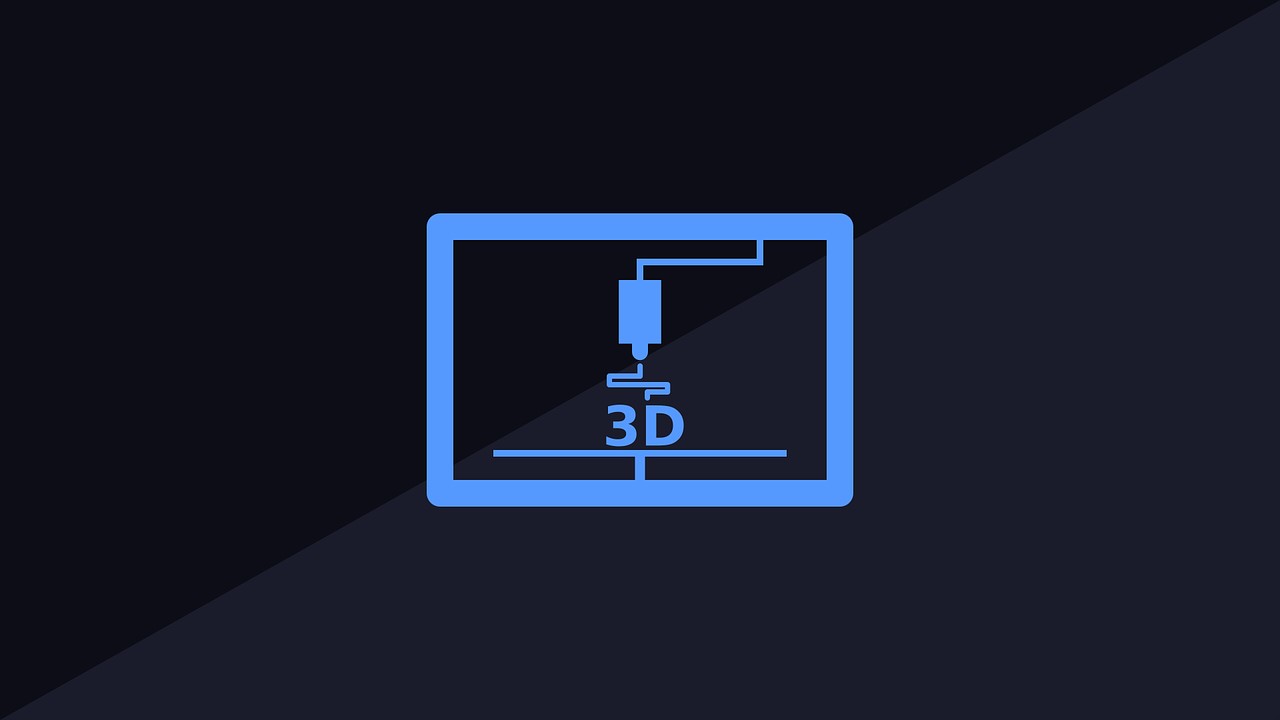
3D bioprinting refers to the 3D printing of living tissue. The process involves either printing living cells within a matrix or printing matrix scaffolds for subsequent addition of cells or in vivo colonisation.
As Nick Leslie, Director of Research at the School of Engineering and Physical Sciences at Heriot-Watt University, puts it “3D bioprinting allows unprecedented spatial control over the 3D organisation of manufactured living material.” Whilst many parameters are optimised for specific applications, current industry research efforts are directed towards the 3D bioprinting of cartilage and bone.
- Understanding Disease Progression with 3D Model Development
- Discussion Group: 3D Model Development
- Human Stem Cell Model Development for Neurodegenerative Diseases
Bioprinting is just one component in a much larger framework of biofabrication - the process of bringing together biological and synthetic materials to create structures with a designed biological function. “Although the process is often conflated specifically with organ printing, which in effect pertains to tissue engineering implants, we are still quite a way off from successfully achieving this,” Kenny Dalgarno, Professor at Newcastle University, explained.
3D Cell Culture Event
Both bioprinting and biofabrication were featured as key topic areas during Oxford Global's recent 3D Cell Culture: Online event on 13th September 2022. The conference program featured over 20 presentations from leading academic and industry representatives working in the field. It showcased some of the cutting-edge research in areas such as 3D cell culture applications in drug screening and therapeutic development.
Two such presentations were delivered by Leslie and Dalgarno, each a highlight of the dedication 3D culture model development track. In this Insight Article, we take an exclusive look at some of the compelling knowledge shared within each academic presentation.
Presentation 1: Bioprinting Technologies for Cancer Models
By Nick Leslie, Director of Research at the School of Engineering and Physical Sciences at Heriot-Watt University
Leslie discussed the utility of suspension printing for delivering cells and extracellular matrices within a suspension volume. “Introducing suspension supports the material during the printing process, which facilitates the use of softer hydrogels to achieve better biological outcomes,” he explained.
Alginate is the most common form of hydrogel used in 3D bioprinting. It is a seaweed-derived polysaccharide that forms cross-links between polymer strands to encapsulate cells within a gel. Alginate is a popular biomaterial for biomedical engineering due to its biocompatibility and ease of use.
Moreover, “the recovery of material from alginate gels can be delivered by calcium chelators which allow for the rapid conversion of gel to fluids, allowing the recovery of cells,” Leslie elaborated. This, in turn, “enables the analysis of dynamic biological phenomena such as protein phosphorylation in a way that is not achievable with many of the other biomaterials that form gels.”
“Printing soft materials can avoid some of the challenges of high-resolution printing without limiting cell behaviour through bioink cross-linking.”
However, one of the most significant drawbacks of using alginate is the potential for dense cross-linking, which can cause limitations to cell behaviour. “And you don't want your cells to be exposed even for short periods of time to a very high concentration of calcium ions,” Leslie explained.
To overcome this, Leslie and his team investigated the use of 3D printing in suspension media, with the aim of facilitating the production of softer hydrogels to allow faster cell migration with better stability. They found that suspension printing in gellan gum and Matrigel enable the deposition of soft cell-laden matrices.
“Printing soft materials can avoid some of the challenges of high-resolution printing without limiting cell behaviour through bioink cross-linking,” Leslie confirmed. Future study aims include characterising ‘Gel-MA in Matrigel' constructs, testing the incorporation of stromal cells with Matrigel baths, and demonstrating the reproducibility of drug sensitivity data.
Presentation 2: Reactive Jet Impingement Bioprinting for High Cell Density Cultures
By Kenny Dalgarno, Professor at Newcastle University
Dalgarno began his presentation by identifying the six steps of biofabrication: imagining, the design approach, material selection, cell selection, bioprinting, and application. The area of biofabrication Dalgarno focused on was the reactive jet impingement process during the bioprinting stage.
“The reactive jet impingement process implemented here at Newcastle University is a way of producing cell-filled gels in a 3D culture,” Dalgarno stated. Compared to other bioprinting methods such as microextrusion, inkjet, and laser-associated technologies, the reactive jet impingement produces higher cell density, providing access to a bone marrow microenvironment much quicker.
Reactive jet impingement creates a gel by reacting a polymer solution together with a cross-linking solution. “We set up two droplet streams with cells in suspension, into two microvalves, with the two droplets meeting mid-air, to react and form a gel,” Dalgarno said. “The reaction is performed at high speed with 200 droplets generated a second” The combined droplet and solution then drops down onto the substrate to create either 2D arrays or 3D structures.
Dalgarno's team use a compound gel consisting of collagen, alginate, and fibrin (or CAF gel), when completing bone micro-tissues. “Alginate and collagen provide an optimal bio-function of quick cross-linking in situ, which is important considering the speed of the reaction,” he explained. This produces a high cell density of 45M cells/ml, meaning “there is more cell than gel,” resulting in more mature tissue. Mechanisms for accelerating the maturation rate of tissues further include fibroblasts in CAF.
Newcastle University is exploring a range of applications for use in developing disease models and early-stage drug screening. Perhaps the most intriguing efforts being made have been those in bone and cartilage constructs. “We are interested in osteoarthritis and the resulting cartilage degradation, and how understanding of the cartilage interface provides a way to assess drug development.” An additional application area is developing a pre-clinical human model of live cancer to test novel therapies, in collaboration with the Newcastle University spin-out, Fibrofind.
What's Next in the Cell Event Series?
Accelerating the adoption of 3D models in pre-clinical and translational research features as a key topic area of our next-in-series event, 3D Cell Culture 2023. Happening from 25th to 26 th April 2023 in London, UK, the programme agenda will be bringing together industry influencers to drive 3D model implementation and development for predictivity, validation, safety, and toxicity studies. This event is co-located with Organ Modelling UK: In-Person.
To register, please click HERE.